S.B.Phillips, W.R. Raun, and G.V. Johnson
Oklahoma State University
Abstract
Nitrate
contamination of groundwater is a growing concern worldwide. Considering that
analytical methods have changed over the past forty years, data collected using
1950's techniques has been incorrectly compared to data collected today. Without
applying random and bias errors associated with each independent method, these
reported changes in NO3-N concentrations are scientifically invalid.
This study was initiated to determine the errors associated with analytical
procedure, seasonal sampling, and storage method for well water NO3-N
analysis. Nitrate-N concentrations for fifty water wells in Grant, Garfield, and
Kingfisher counties, obtained during the period 1950-1972, were acquired through
cooperation with The United States Geological Survey and The Oklahoma Water
Resources Board. Well water samples were taken at different dates from these
same locations in 1993 and 1994. For each sampling date, four samples were taken
from each well whereby two were frozen immediately (as per EPA protocol), and
two were stored at ambient temperature for five to ten days as was common in
1950. Water samples were then analyzed using phenoldisulfonic acid (1950) and
automated cadmium reduction(1993) procedures. Mean NO3-N values from
analysis using cadmium reduction were significantly higher than values obtained
using the phenoldisulfonic acid procedure, reflecting the bias associated with
making direct numerical comparisons between procedures. Comparisons made between
1950 and 1993 well water analysis (employing the same phenoldisulfonic acid
procedure) indicate that few significant increases have taken place over the
past forty years. No relationship between well water NO3-N and depth
to aquifer was found which indirectly suggests that NO3-N leaching
was not significant for this population of 50 wells.
Introduction
Nitrate-N
contamination of groundwater is a growing concern worldwide. When
nitrate-nitrogen(NO3-N) is ingested by infants, nitrate can be
reduced to nitrite. Nitrite-N can occupy sites on hemoglobin that would normally
carry oxygen. This reduced oxygen carrying capacity of the blood is what leads
to methemoglobinemia, or "blue baby" syndrome which can be fatal to infants
under six months of age. This condition is, however, treatable and fatalities
are rare. In the last thirty years, only one death in the United States was
linked to Nitrate poisoning caused from drinking well water (Fedkiw, 1991). The
maximum level for NO3-N in drinking water is 10 mg/kg(as set by the
Environmental Protection Agency). Results from a national survey (National
Pesticide Survey, 1990) noted that only a small percentage (2.4%) of rural water
wells exceed this level.
The issue
that groundwater NO3-N concentrations have increased over time is not
disputed. Nitrate-N occurs naturally in the soil (Johnson, 1993). Despite this
fact, agricultural chemicals, particularly N fertilizers, have received the bulk
of the blame for the increase. A computer based literature search performed by
L.W. Canter found 34 references where groundwater NO3-N was
associated with fertilizer use (Canter, 1987). In order for fertilizers to be
directly responsible for groundwater contamination, NO3-N would have
to be leached out of the soil profile. The occurrence of this is preceded by
other processes resulting in much higher levels of N removal (Mills et al.,
1974; Sharpe et al., 1988; Hooker et al., 1980; Aulakh et al., 1984). DeWalle
and Schaff, 1980, Hallberg, 1983, and Olsen ,1974, among many others, have
reported agriculture related increases in groundwater NO3-N
concentrations over time periods of up to thirty years. Considering that
analytical methods have changed since the 1950's, direct numerical comparison
between data obtained thirty years ago and data obtained today is erroneous.
Schepers et al., 1991, Walters and Malzer, 1990, and Webster et al., 1986, have
also evaluated the impacts of N fertilizers without addressing the analytical
errors associated with these estimates. Without adequately assessing the random
and bias errors associated with an independent estimate, researchers are at risk
of making scientifically invalid conclusions about changes in NO3-N
concentrations.
The
objective of this research was to determine the errors associated with
analytical procedure, seasonal sampling, and storage method for well water NO3-N
analysis. A thorough understanding of the errors associated with estimates of NO3-N
in groundwater will assist researchers in identifying where significant
increases have taken place.
Materials and Methods
Fifty
water wells in Garfield, Grant, and Kingfisher counties (Figure 1) were
selected for comprehensive sampling. These wells were selected on the basis that
NO3-N data collected during the time period 1953-1972 was available
(Bingham and Bergman, 1980; Dover, 1953; U.S.G.S., 1993). These counties also
have substantial agricultural activity associated with continuous wheat
production and N fertilization. Locations and physical characteristics for each
well site are found in Table 1. Tax records obtained from the three counties
were used to determine current ownership of the property on which each well was
located. The owners were contacted and informed about the experiment. Permission
was obtained from the well owners to begin quarterly well water sampling
beginning in the fall of 1993.
In order
to obtain a representative groundwater sample, it is desirable to take the
sample directly from the aquifer. However, 39 of the 50 wells contain in-place
semi-permanent mounted pumps which limit the options available for groundwater
sampling. These wells were pumped for 5-10 minutes prior to sampling so that
water in the well reasonably represented that of the aquifer. Of the 11 other
wells, 7 were collected via windmills and 4 were collected using a teflon
bailer. All samples were taken using proper sampling protocol (Barcelona et al.,
1987; Davis et al., 1993; Scalf et al., 1981). For each sampling date, four
samples were taken from each well whereby two were frozen immediately (AOAC,
1984) by being placed in a cooler containing dry ice and two were stored at
ambient temperatures for five to ten days as was common in 1950.
Frozen
and non-frozen samples were analyzed using two methods. One method was
phenoldisulfonic acid (Bremner, 1965; Chapman and Pratt, 1961; Snell, 1949), a
procedure commonly used in 1950 when the original NO3-N data was
collected. The other method, automated cadmium reduction (Henriksen and
Selmer-Olsen, 1970; Jackson et al., 1975), was performed using the
Lachat-Quickchem automated flow injection system (1993). Statistical analysis
of data was performed using procedures outlined by the SAS institute (SAS,
1988). Geographic Information Systems was used to produce surface and contour
maps showing relationships among wells and analytical methods.
Results and Discussion
Significant differences (.0001 probability) in well water NO3-N were
found between season of sampling and method of analysis. The mean NO3-N
value for samples taken in each of the four seasons ranged from 6.97 ± 5.8 in
the fall to 9.59 ± 8.65 in the summer. This agrees with work done by Gilliam et
al., 1974, Hallberg et al., 1983, and McDonald and Splinter, 1982, all of whom
found NO3-N levels for a specific well to vary among seasons. This
reflects a bias error associated with seasonal sampling. The difference between
frozen and non-frozen samples was statistically insignificant (.8415
probability). The largest difference in means was found when comparisons were
made between analytical methods. Mean well water NO3-N concentrations
found by using the phenoldisulfonic acid procedure were 3.2 mg/kg lower than
those found using cadmium reduction (9.4 ± 7.5 vs 6.2 ± 4.7). Possible
explanations for this difference are that a loss of NO3-N occurred
when using the phenoldisulfonic acid procedure, or that the accuracy of
phenoldisulfonic acid is poor in terms of NO3-N detection. In either
case, an error exists. Comparing population means generated by all possible
combinations of the variables (season, storage, and method) resulted in
overlapping standard deviations which seriously restricted whether or not
conclusive changes had taken place when comparing data obtained using
independent methods (Figure 2). Comparisons made between 1950 and 1993 well
water analysis (employing the same phenoldisulfonic acid procedure) indicated
that 57 % of all wells sampled have shown either a significant decrease or no
significant change at all over the past forty years (Table 2).
No
relationship was found between the average depth to aquifer and well water NO3-N
in either 1953, 1993 or the difference (1993-1953, Figure 3). Because
fertilizer use was not common before 1950, increased fertilizer use from
1950-present should have resulted in significant well water NO3-N
increases in more shallow aquifers if in fact NO3-N leaching from
surface applied fertilizers was present. If NO3-N leaching from the
excessive use of N fertilizers were to have been significant over this forty
year time period, depth to the aquifer (on these extremely shallow wells, <30
ft) and well water NO3-N should have been negatively correlated.
References
Aulakh,
M.S., D.A. Rennie, and E.A. Paul. 1984. The influence of plant residues on
denitrification rates in conventional and zero tilled soils. Soil Sci. Soc. Am.
J. 48:790-794.
Bremner, J.M. 1965. Inorganic forms of nitrogen. In C.A. Black et al. (ed.)
Methods of soil analysis, Part 2. Agronomy 9:1179-1237. Am. Soc. of Agron.,
Inc., Madison, WI.
Canter,
L.W. 1987. Nitrates and Pesticides in Groundwater: An Analysis of a Computer
Based Literature Search. In Deborah M. Fairchild (ed.) Groundwater
Quality and Agricultural Practices. Lewis Publishers. Chelsea, MI.
Dewalle,
F.B., and R.M. Schaff. 1980. Groundwater pollution by septic tank drainfields.
J. Environmental Engineering Division. 106(EE3):631-648.
Dover,
T.B. 1953. Chemical character of public water supplies in Oklahoma, 1953.
Bulletin No. 8. Division of Water Resources, Oklahoma Planning and Resources
Board. U.S. Geological Survey.
Fedkiw,
John. 1991. Nitrate occurrence in U.S. waters, a reference summary of published
sources from an agricultural perspective. United States Department of
Agriculture, Washington DC.
Hallberg,
G.R. et al. 1983. Hydrogeology, Water Quality , and Land Management in the Big
Spring Basin, Clayton County, Iowa. Open file report No. 83-3. Iowa Geological
Survey. Iowa City, Iowa.
Henriksen, H., and A.A. Selmer‑Olsen. 1970. Automatic methods for determining
nitrate and nitrite in water and soil extracts. Analyst 95:514‑518
Hooker
M.L., D.H. Sander, G.A. Peterson, and L.A. Diagger. 1980. Gaseous N losses from
winter wheat. Agron. J. 72:789-792.
Jackson, W.A., C.E. Frost, and D.M. Hildreth. 1975. Versatile multi‑range
analytical manifold for automated analysis of nitrate‑N. Soil Sci. Soc. Am.
Proc. 39:592‑593
Johnson,
G.V. 1993. Fate of Fertilizer Nitrogen in Soils. Environmental Notes. Oklahoma
State University Center for Agriculture and the Environment, Stillwater, OK.
Milham,
P.J., A.S. Awad, r.E. Paull, and J.H. Bull. 1970. Analysis of plants, soils and
waters for nitrate by using an ion-selective electrode. Analyst. 95:751-757.
Mills,
Harry A., Allen V. Barker, and Donald N. Maynard. 1974. Ammonia volatilization
from soils. Agron. J. 66:355-358.
National Research Council. Committee on Nitrate Accumulation. 1972. Fertilizer
and Soil Nitrogen. p. 40. In: Accumulation of Nitrate. National Academy
of Sciences. Washington, D.C.
Official Methods of Analysis of the Association of Official Analytical Chemists.
1984. Association of Official Analytical Chemists, Inc., Arlington, VA.
Patterson, H.D., and B.I. Lowe. 1970. The errors of long-term experiments. J.
Agric. Sci. (Cambridge) 74:53-60.
Schepers, J.S., M.G. Moravek, E.E. Alberts, and K.D. Frank. 1991. Maize
production impacts on groundwater quality. J. Environ. Qual.
20:12-16.
Sharpe,
R.R., L.A. Harper, J.E. Giddens, and G.W. Langdale. 1988. Nitrogen use
efficiency and nitrogen budget for conservation tilled wheat. Soil Sci. Soc. Am.
J. 52:1394-1398.
Snell,
F.D., and C.T. Snell. 1949. Colorimteric methods of analysis, Vol. 2. 3rd ed. D.
Van Nostrand Co., New York.
United
States Department of Agriculture Extension Service. 1990. National Pesticide
Survey; Phase One Report. United States Department of Agriculture, Washington
DC.
Walters, D.T., and G.L. Malzer. 1990. Nitrogen management and nitrification
inhibitor effects on nitrogen-15 urea: II. Nitrogen leaching and balance. Soil
Sci. Soc. Am. J. 54:122-130.
Webster, C.P., R.K. Belford, and R.Q. Cannell. 1986. Crop uptake and leaching
losses of 15N labeled fertilizer nitrogen in relation to waterlogging
of clay and sandy loam soils. Plant Soil 92:89-101.
Table 1. Location, soil type,
major land use and average depth to aquifer for each well sampled in 1953 and
1993, Grant, Garfield and Kingfisher Counties, OK.
WELL |
COUNTY |
LOCATION |
SOIL
TYPE |
MAJOR
LAND USE |
AVG.
DEPTH TO AQUIFER |
|
|
|
|
|
IN FEET
BELOW SURFACE |
1 |
KINGFISHER |
15N-07W-02 CC |
KINGFISHER SILT LOAM, 3 TO 5 % SLOPE |
CROPLAND |
10 |
2 |
KINGFISHER |
16N-07W-29 C |
RENFROW
CLAY LOAM, 1 TO 3 % SLOPE |
CROPLAND |
9 |
3 |
KINGFISHER |
17N-07W-36 B |
NORGE
FINE SANDY LOAM, 0 TO 1 % SLOPE |
CROPLAND |
19 |
4 |
KINGFISHER |
17N-06W-31 B |
PORT
SILT LOAM, 0 TO 1 % SLOPE |
RANGELAND |
19 |
5 |
KINGFISHER |
17N-05W-31 D |
KINGFISHER SILT LOAM, 3 TO 5 % SLOPE |
CROPLAND |
35 |
6 |
KINGFISHER |
17N-05W-32 B |
PORT
CLAY LOAM, 0 TO 1 % SLOPE |
CROPLAND |
35 |
7 |
KINGFISHER |
17N
-05W-32 C |
PORT
CLAY LOAM, 0 TO 1 % SLOPE |
CROPLAND |
35 |
8 |
KINGFISHER |
17N-05W-35 D |
PRATT
LOAMY FINE SAND, UNDULATING |
CROPLAND |
22 |
9 |
KINGFISHER |
17N-05W-36 A |
PRATT
LOAMY FINE SAND, UNDULATING |
CROPLAND |
27 |
10 |
KINGFISHER |
17N-05W-18 D |
PRATT
LOAMY FINE SAND, HUMMOCKY |
CROPLAND |
25 |
11 |
KINGFISHER |
17N-07W-11 D |
LINCOLN
LOAMY FINE SAND |
IRRIGATED PASTURELAND |
10 |
12 |
KINGFISHER |
17N-08W-4 C |
NORGE-SLICKSPOT COMPLEX, 1 TO 3 % SLOPE |
CROPLAND |
7 |
13 |
KINGFISHER |
17N-07W-06 B |
NORGE
FINE SANDY LOAM, 0 TO 1 SLOPE |
CROPLAND |
5 |
14 |
KINGFISHER |
17N-07W-01 C |
PRATT
LOAMY FINE SAND, HUMMOCKY |
RANGELAND |
7 |
15 |
KINGFISHER |
18N-06W-32 B |
PRATT
LOAMY FINE SAND, UNDULATING |
CROPLAND |
28 |
16 |
KINGFISHER |
18N-07W-28 C |
PRATT
LOAMY FINE SAND, UNDULATING |
CROPLAND |
11 |
17 |
KINGFISHER |
18N-07W-24 A |
DOUGHERTY-EUFALA LOAMY FINE SAND, HUMMOCKY |
IRRIGATED CROPLAND |
29 |
18 |
KINGFISHER |
18N-07W-13 AC |
CARWILE
LOAMY FINE SAND |
CROPLAND |
25 |
19 |
KINGFISHER |
18N-07W-15 A |
SHELLABARGER FINE SANDY LOAM, 5 TO 8 % SLOPE |
RANGELAND |
26 |
20 |
KINGFISHER |
18N-07W-08 D |
DOUGHERTY-EUFALA LOAMY FINE SAND, UNDULATING |
CROPLAND |
26 |
21 |
KINGFISHER |
18N-08W-02 D |
PRATT
LOAMY FINE SAND, HUMMOCKY |
CROPLAND |
18 |
22 |
KINGFISHER |
18N-O8W-02 C |
DOUGHERTY-EUFALA LOAMY FINE SAND, HUMMOCKY |
PASTURELAND |
18 |
23 |
KINGFISHER |
19N-07W-29 D |
DOUGHERTY-EUFALA LOAMY FINE SAND, UNDULATING |
CROPLAND |
33 |
24 |
KINGFISHER |
19N-07W-8 D |
CARWILE
LOAMY FINE SAND |
CROPLAND |
6 |
25 |
KINGFISHER |
19N-08W-11 DA |
DOUGHERTY-EUFALA LOAMY FINE SAND, HUMMOCKY |
IRRIGATED PASTURELAND |
13 |
26 |
KINGFISHER |
19N-08W-11 A |
DOUGHERTY-EUFALA LOAMY FINE SAND, HUMMOCKY |
IRRIGATED PASTURELAND |
13 |
27 |
KINGFISHER |
15N-07W-02 C |
ALLUVIAL
AND BROKEN LAND |
CROPLAND |
7 |
28 |
KINGFISHER |
19N-09W-23 BBB |
EUFALA
FINE SAND |
CROPLAND |
11 |
29 |
KING
FISHER |
19N-09W-10 AB |
PRATT
LOAMY FINE SAND, UNDULATING |
RANGELAND |
17 |
30 |
KINGFISHER |
19N-08W-05 B |
DOUGHERTY-EUFALA LOAMY FINE SAND, UNDULATING |
CROPLAND |
8 |
31 |
GARFIELD |
20N-08W-23 CC |
SHELLABARGER FINE SANDY LOAM, 1 TO 3 % SLOPE |
CROPLAND |
20 |
32 |
GARFIELD |
20N-05W-28 A |
PORT
CLAY LOAM |
CROPLAND |
18 |
33 |
GARFIELD |
20N-03-W-26 BBC |
ERODED
CLAYEY LAND |
RANGELAND |
38 |
34 |
GARFIELD |
21N-04W-11 D |
KIRKLAND
SILT LOAM, 0 TO 1 % SLOPE |
CROPLAND |
19 |
35 |
GARFIELD |
21N-06W-12 CCB |
PORT
SILT LOAM, 0 TO 1 % SLOPE |
CROPLAND |
18 |
36 |
GARFIELD |
21N-07W-23 BBC |
POND
CREEK SILT LOAM, 0 TO 1 % SLOPE |
CROPLAND |
18 |
37 |
GARFIELD |
21N-08W-20 CCC |
DRUMMOND
SOILS |
RANGELAND |
11 |
38 |
GARFIELD |
22N-O8W-18 A |
GRANT-NASH SILT LOAM, 5 TO 8 % SLOPE |
RANGELAND |
3 |
39 |
GARFIELD |
22N-07W-01 D |
SHELLABARGER-CARWILE FINE SANDY LOAM, UNDULATING |
URBAN/BUILT UP LAND |
18 |
41 |
GARFIELD |
24N-03W-05 B |
RENFROW-VERNON COMPLEX, 3 TO 5 % SLOPE, ERODED |
CROPLAND |
10 |
42 |
GARFIELD |
24N-05W-03 C |
KIRKLAND-RENFROW SILT LOAM, 1 TO 3 % SLOPE |
CROPLAND |
18 |
43 |
GARFIELD |
24N-05W-35 A |
KIRKLAND
SILT LOAM, 0 TO 1 % SLOPE |
CROPLAND |
17 |
44 |
GARFIELD |
23N-06W-7 D |
MENO
LOAMY FINE SAND, UNDULATING |
RANGELAND |
20 |
45 |
GARFIELD |
23N-07W-7 C |
POND
CREEK SILT LOAM, 0 TO 1 % SLOPE |
CROPLAND |
27 |
46 |
GARFIELD |
24N-07W-21 ADD |
GRANT
SILT LOAM, 3 TO 5 % SLOPE, ERODED |
CROPLAND |
18 |
48 |
GRANT |
25N-05W-6 D |
YAHOLA
FINE SANDY LOAM, OCCASIONALLY FLOODED |
RANGELAND |
10 |
49 |
GRANT |
28N-07W-24 A |
QUINLAN-WOODWARD LOAM, 3 TO 12 % SLOPE |
RANGELAND |
6 |
50 |
GRANT |
27N-03W-28 B |
RENFROW
SILTY CLAY LOAM, 2 TO 5 % SLOPE, ERODED |
CROPLAND |
26 |
51 |
GRANT |
27N-03W-22 B |
McLAIN-DRUMMOND
SILT LOAM, RARELY FLOODED |
RANGELAND |
30 |
52 |
GRANT |
27N-03W-23 A |
KIRKLAND
SILT LOAM, 1 TO 3 % SLOPE |
CROPLAND |
30 |
|
|
|
|
|
|
Table 2.
Nitrate-N concentrations for each well sampled between 1950 and 1972 and the
1993 sampling data analyzed using the phenoldisulfonic acid procedure when
samples were not frozen, Grant, Garfield and Kingfisher Counties, OK.
______________________________________________________________________________
1993
1950-72
Well
No. NO3-N
SD NO3-N
Sig.
______________________________________________________________________________
3
8.80 0.20
0.02
**
4
2.90 0.16
42.70
**
5
9.06
0.10
.11
**
6
2.52
0.16
.18 ns
7
12.20
0.06
.02
**
8
14.60
0.03
.14
**
9
8.24 0.06
6.75 ns
10
7.61 0.10
8.78 ns
11
0.45 0.13
0.00 ns
12
10.00 0.25
0.16
**
14
12.40 0.16
0.29
**
15
6.76
0.41
. ns
16
11.10 0.65
11.30 ns
17
18.20
0.76
. ns
18
11.90 0.98
5.85
**
19
10.00 0.03
36.00
**
20
7.89 0.02
3.83
**
21
3.04 0.30
0.29
*
22
6.54
0.36
. ns
23
7.10 0.30
2.70
**
24
12.00 2.21
1.31
**
25
13.40 0.10
67.50
**
26
12.50 0.92
38.30
**
29
5.38 0.13
4.50 ns
31
15.20 0.79
5.85
**
32
2.33 0.12
0.77 ns
34
5.78 0.05
1.91
**
35
6.41 0.01
2.00
**
36
3.58 0.03
1.13 ns
37
5.38 0.00
1.24
**
38
1.19 0.18
22.50
**
41
14.30 0.21
6.98
**
42
0.61 0.40
6.75
**
43
1.02 0.21
0.38 ns
44
11.40 0.36
2.25
**
45
5.17 0.17
1.76
*
46
2.65 0.03
3.38 ns
48
1.98 0.25
0.02 ns
49
1.26 0.54
1.91 ns
51
6.97 0.46
13.50
**
52
0.54 0.31
5.85
**
______________________________________________________________________________
**, *
-
significant decrease at 0.01 and 0.05 probability levels respectively.
**, *
-
significant increase at 0.01 and 0.05 probability levels respectively.
ns - not significant.
43% significant increase
20% significant decrease
37% no significant
change
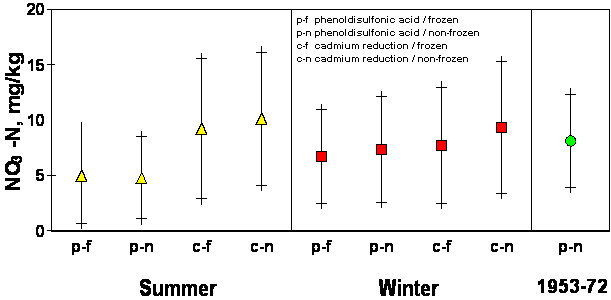
Figure 2.
Nitrate-N analysis by season, analytical procedure and storage method from well
water samples collected in Grand, Garfield and Kingfisher Counties, OK,
1953-1993.
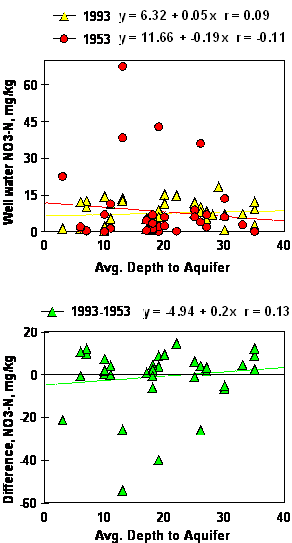
Figure 3.
Simple linear regression of well water NO3-N on the average depth to aquifer,
1993, 1953 and the difference between 1993 and 1953.
|