R.K. Teal, K.W. Freeman, K. Girma, D.B. Arnall,
J.W. Lawles, K.L. Martin, R.W. Mullen, and W.R. Raun
(accepted J. of Sustainable Agriculture)
044 AgHall, Department of Plant and Soil Sciences, Oklahoma State University,
Stillwater, OK 74078
Correspondence: William R. Raun, 044 North Ag
Hall, Department of Plant and Soil Sciences, Oklahoma State University, OK
74078; Telephone: (405) 744 6418; Fax: (405) 774 5269; E-mail: wrr@mail.pss.okstate.edu
ABSTRACT
Nitrogen use
efficiency (NUE) in cereal grain production is estimated to be 33%
throughout the world, and can be lower when N is applied in single,
pre-plant applications compared with split N applications. This study was
conducted to evaluate tillage system and anhydrous ammonia (AA) application
methods on yield, N uptake, and NUE in hard red winter wheat (Triticum
aestivum L.), using a narrow (10 cm) nozzle spacing on a V-blade (Noble
or sweep blade) applicator and wide (46 cm) nozzle spacing on a knife
applicator. Over the four-year period evaluated, conventional tillage was
significantly higher in grain yield in five of eight site years over
no-till. However, no-till was significantly higher in grain yield at Lahoma
in 2003 where the highest overall grain yield was observed. Mixed results
were evaluated in NUE for tillage; four site years of no significant
differences between tillage systems and the other four site years split
evenly between conventional till and no-till. The V-blade improved NUE in
no-till for three site years at Lahoma, while the knife applicator increased
NUE the initial year at Efaw in no-till. Previous crop residue disturbance
averaged less than 15% for both AA applicators all four, site years.
Mid-season plant populations taken during the 2003 and 2004 crop years were
insignificant three of the four site years and plant population did not
influence grain yield and NUE. No-till crop production reduced soil
compaction at Efaw and the V-blade applicator reduced soil compaction within
no-till at both locations. Although the no-till system showed the potential
to produce grain yield and grain N levels comparable to conventional
tillage, conventional tillage had a distinct advantage in grain yield and
grain N uptake over the four-year duration of this study. The V-blade
application method improved NUE in no-till at one site, potentially due to
reduced soil compaction, but neither AA applicator showed an advantage in
conventional tillage. Over the four years of this study no-till reduced
soil compaction and conventional tillage produced higher grain yields, but
no conclusive advantages were found in NUE for either tillage practice or AA
application method.
Keywords:
no-till, v-blade, nitrogen, anhydrous ammonia, winter wheat, NUE
INTRODUCTION
Soil erosion has been a
major concern since the beginning of agriculture, but it was not until the
Great Dust Bowl of the 1930s that the problem received worldwide attention.
With so many deaths caused by black pneumonia and total crop destruction by
wind-borne soil in massive volumes, measures were taken to make sure that
this would never happen again. Zero tillage or No-till was originally used
as a method to stop soil erosion. McGregor et al. (1992) reported that
during a 5-year period (1987 through 1991), no-till soybean yielded 44% more
than conventional-till yields. Intensive tillage has led to annual sediment
discharge of 15.9 Mg ha-1 in the southern Great Plains (Smith et
al., 1991). Work by McGregor et al. (1992) showed increasing soil losses
with time under conventional-till and decreasing soil loses with time for
no-till. This continued study conducted over 14 years; noted no-till
soybean yields exceeded those of conventional-till yields by 800 kg ha-1
yr-1 (McGregor et al., 1999). No-till reduced runoff 1 to 35%
over conventional-till and reduced soil loss by 23 to 77% compared to
conventional-till (McGregor et al., 1999). King et al. (1995) similarly
found that long-term no-till practices are effective and practical in
reducing rill erodibility and sediment loss.
There have been several
other unforeseen advantages of no-till over conventional-till that
researchers have discovered over time. Edwards et al. (1990) found that
no-till improved soil drainage, while Weersink et al. (1992) stated that
no-till reduces labor costs. Aase and Pikul (1995) found that in annual
spring wheat production, no-till was the most efficient crop and soil
management practice from the standpoint of yield, water use efficiency, soil
organic C, and bulk density. However, many researchers have reported that
bulk density increases under no-till versus conventional-till (Vyn and
Raimbault, 1993), as well as that tillage has no effect on bulk density (Ismail
et al., 1994). Alternatively, Lal et al. (1994) and Dao (1993) supported
studies that reported no-till reduced bulk density. There have been other
controversies comparing no-till to conventional-till systems as well as bulk
density, one such argument being pH. Dick (1983) found that pH decreased
under no-till as compared to conventional-till as nitrogen rates were
increased, but Lal et al. (1994) found no effect of tillage on pH.
Research
has indicated that soil organic matter content is related to amount of
residue returned to the soil (Eghball et al., 1994; Christensen et al.,
1994). Loss of soil organic C and N in the Great Plains has been caused by
the use of tillage and summer fallow, which have accelerated organic matter
decomposition rates and erosion. Ismail et al. (1994), Lal et al. (1994),
and Christensen et al. (1994) reported that soil organic matter was greater
under no-till and increased with time in some instances. Bauer and Black
(1994) discovered that 1 Mg ha-1 of soil organic matter
contributed the equivalent of 15.6 kg ha-1 of wheat grain yield.
Further comparisons showed that no-till enhanced N immobilization and
reduced nitrification rates when compared to conventional-till (Doran,
1980), often resulting in less nitrate leaching and leaving less nitrate in
the soil profile (Lamb et al., 1985). Although there are lower nitrate
levels in soil profiles in no-till systems, studies have shown that nitrate
has been found deeper in the profiles of no-till soils (Eck and Jones,
1992).
The results
of a 10-year study showed that N-mineralization rates were higher in annual
cropping systems under no-till, than under conventional-till (Wienhold and
Halvorson, 1999). Increased N stored as labile organic forms causes this
increased mineralization. Increased amounts of organic N will supply more
nitrogen to crops, which will result in less N required from fertilizers as
well as reduced leaching. Wienhold and Halvorson (1999) also believe that
higher N rates will increase immobilization because of the increased plant
residue resulting from the higher N rates increasing the C/N ratio of the
residue. Other studies have shown that immobilization was higher at lower
applied N rates and crop N uptake was less with no-till systems (Smith and
Sharpley, 1990; Knowles et al., 1993). Their research has also found
evidence that immobilization of surface applied N fertilizers accounts for
most of the differences in N response between no-till and conventional-till
systems. Their research also shows that no-till systems required more N
fertilizer when surface applied at lower rates. However, Fox and Bandel
(1986) discovered that no-till increased mineralization compared to
conventional-till during the latter part of the growing season. Rodriguez
and Giambiagi (1995) found that no-till enhances denitrification, because of
the increase in soil water supply commonly occurring in no-till, reducing
the amount of aerobic activity in the soil. There are some conflicting
views between Wienhold and Halvorson (1999) and the others stated above, but
keep in mind that the Wienhold and Halvorson (1999) study was long-term (10
years), while the others were short-term (5 years or less). Wienhold and
Halvorson were the only ones to account for the build up of soil organic
matter (OM), and it would not be possible for soil OM to be a major factor
in a short-term study.
Bonfil et
al. (1999) found that no-till management over a 5-year study increased
yields 62 to 67% in wheat-fallow rotations and 18 to 75% in continuous wheat
over conventional-till in semiarid regions of Israel. Cantero-Martinez et
al. (1999) and Peterson et al. (1996) found similar results in Australia and
the Great Plains of the United States. No-till increases soil water
absorption by reducing evaporation, increasing water infiltration, improving
soil structure, which in turn enhances root development (Norwood, 1994;
Merrill et al., 1996; Dao, 1993). Winter wheat is now being produced
successfully and out-yielding spring wheat in the Northern Great Plains of
the United States and Canada without requiring a fallow period, when no-till
is used with adequate N fertilization (Halvorson et al., 1999). By
increasing stored water in the soil, no-till has reduced the detrimental
effects of climate variability on annual winter wheat production (Dao,
1993).
Studies
have shown that deep placement of N can minimize volatilization losses and
immobilization. Placement of N is a major factor of N utilization and a 20%
increase in NUE has been observed with band placement, compared to surface
broadcast (Tomar and Soper, 1981). They found that reduced N immobilization
and increased N uptake could be achieved by reducing fertilizer contact with
the surface residue. Rao and Dao (1996) found that final grain yield and
grain N content were not affected by N placement in plowed plots. No-till
improved grain yield by 32% for a below the seed row (BL) application and
15% for between the rows (BT) application. Grain N content was increased by
33% for BL and 25% for BT as compared to a surface broadcast application.
Surface applications of
ammonium-based N can be lost to the atmosphere up to 70% from volatilization
(Hamid and Mahler, 1994) and nitrate more readily leaches from the soil than
ammonium; therefore, injected ammonium-based N has the greatest potential to
increase NUE in single pre-plant applications. Some researchers have agreed
that AA moves more in sandy soils with low CEC and low moisture than finer
textured soils with high CEC, but under moist conditions and at depths over
10 cm, high rates of AA can be applied with little or no loss from
volatilization (Swart et al., 1971). Swart et al. (1971) found that ammonia
losses were reduced considerably when the applications were changed from 102
cm to 41 cm spacing (greater yields and less ammonia loss at 41 cm), but no
differences between 15 cm and 41 cm. Swart et al. (1971) went on to report
while vertical movement remains constant (4 to 5 cm) regardless of N rate,
higher N rates usually cause greater lateral movement. Other research has
suggested that AA decreases pH and depletes the amount exchangeable Ca and
Mg leading to decreases in yield due to higher levels of aluminum
accumulation (Bouman et al., 1995). Since AA is the cheapest form of
commercial inorganic N fertilizer and most Oklahoma soils allow for deep
injection, it is a widely used N fertilizer in Oklahoma accounting for 21%
(24,483 Mg) of all applied N fertilizer in the 2004-05 crop year (Oklahoma
Department of Agriculture, 2005, personal communication).
The objectives of this experiment were to determine the
effects of tillage and AA application rate and placement on grain yield and
NUE of hard red winter wheat.
MATERIALS AND METHODS
Two
experimental sites were established in the fall of 2000, one near
Stillwater, OK at the Agronomy Research Station (Easpur loam fine-loamy,
mixed, superactive, thermic Fluventic Haplustoll), and one in Lahoma, OK at
the North-Central Oklahoma Research Station (Grant silt loam fine-silty,
mixed, thermic Udic Argiustoll). Initial soil test results are reported in
Table 1. The experiment employed an N rate by N method factorial
arrangement in a thrice replicated, randomized complete block design within
each level of tillage. Individual plots measured 3.0 x 4.6 m.
Anhydrous
ammonia (82-0-0) was applied at rates of 0, 61, 123, and 185 kg N ha-1
using two different methods of injection. A rolling coulter applicator
(DMI) with five knives spaced 46 cm apart at a depth of 15 cm, a method
commonly used in nitrogen application of winter wheat, was used as one
method of AA application. The Noble or undercutting blade (V-Blade), an
experimental applicator, was used as the other method of AA application.
The V-blade applicator has a single coulter, centered in front of the point
of the undercutting blade, where AA was applied in 10 cm bands at a depth of
10 cm and a total width of 1.5 meters.
The winter wheat
variety ‘Jagger’ was planted at both sites (planting and fertilizer dates
are reported in Table 2). At the Lahoma site, a seeding rate of 95 kg ha-1
was planted the initial year and increased to 125 kg ha-1 the
second year and was sustained through the third and fourth years in 19 cm
rows within wheat stubble from the previous year as well as conventionally
worked ground. Triple super phosphate (0-46-0) was applied pre-plant at a
rate of 39 kg P ha-1 the first two years at Lahoma to alleviate
possible phosphorous deficiencies, however starting in 2002 at both
locations, triple super phosphate was banded with the seed at a rate of 12
kg P ha-1. At the Efaw site, a seeding rate of 125 kg ha-1
was planted in 15 cm rows in grain sorghum stubble from the previous summer
as well as conventionally worked ground the first two years, but beginning
in 2002 the same seeding rate was planted in 18 cm rows within wheat stubble
from the previous year as well as conventionally worked ground. In this
case, conventional tillage at both sites consisted of disking throughout the
summer, and preparing the seedbed with a field cultivator.
Wheat residue cover was measured before and after AA
applications on the no-till starting in 2002, using a modified,
line-intercept method (Morrison et al., 1997) as a means of determining
residue disturbance (residue disturbance = residue cover (%) before AA –
residue cover (%) after AA) by AA application method.
In addition,
plant counts were taken from a 0.54 m2 area
out of the center of each plot for evaluation of plant stands as a potential
independent variable or covariate beginning in 2002. Furthermore, bulk
density measurements using a cylinder (7.5 x 15 cm) and soil compaction
measurements using an electronic cone penetrometer (SC 900 Soil Compaction
Meter, Spectrum Technologies, Inc.) at 2.5 cm intervals, to a depth of 30 cm
were taken post-harvest from each plot in 2004.
Wheat grain was
harvested with a Massey Ferguson 8XP experimental combine, removing an area
of 2.0 x 4.6 m from the center of each plot. A Harvest Master
yield-monitoring computer installed on the combine was used to record yield
data. Grain yield from each plot was determined and a sub-sample was taken
for total N analysis. Grain samples were dried in a forced air oven at 66oC,
ground to pass a 140 mesh sieve (100 um), and analyzed for total N content
using a Carlo-Erba NA 1500 automated dry combustion analyzer (Schepers et
al., 1989). Using the difference method, NUE was calculated by subtracting
the grain N uptake of the 0 N plot from the N fertilized plot grain N
uptake, then dividing by the N rate applied to the N fertilized plot.
Analyses of variance and single degree of freedom contrasts were performed
using SAS (SAS, 1990).
RESULTS AND DISSCUSION
Grain Yield
Delayed
planting due to inclement weather at planting time (Table 2) resulted in
poor establishment and limited tillering and wheat yield responses to
applied N were minimal in 2001 at both locations. However, grain yields
were much higher in all three of the following years, particularly in 2003
and 2004 when response to applied N was high. While there were no
differences between tillage systems at Efaw in 2001 and 2004, conventional
tillage did result in significantly higher grain yields at Efaw in 2002 and
2003 (Table 3). Similar advantages in grain yield were observed for
conventional tillage in 2001, 2002, and 2004 at Lahoma, but in 2003 the
no-till treatments had significantly higher grain yields with three out of
eight treatments averaging 5 Mg ha-1 (Table 4).
Grain yield
was significantly affected by N application method for the Lahoma no-till in
2002 when the V-blade applicator increased yield over the knife applicator
(P < 0.001), otherwise there was no significant difference between the
applicators on grain yield for either tillage system at either location. At
Efaw a positive linear response to N using both applicators was observed in
the no-till treatments in all four years (P < 0.05). A positive linear
trend was also observed at Efaw in the conventional till treatments with the
knife applicator in 2001 (P < 0.1) and 2004 (P < 0.001) and with the V-blade
applicator in 2001 (P < 0.01). A quadratic grain yield response to N
occurred with the V-blade applicator for conventional till in 2003 (P <
0.01) and 2004 (P < 0.01).
At Lahoma a positive
linear grain yield response to N was observed with the knife applicator for
no-till in 2001 (P < 0.05) and 2004 (P < 0.01) and for conventional till in
2003 (P < 0.1). Highly significant quadratic grain yield responses to N
rate occurred at Lahoma with the knife applicator for no-till in 2002 (P <
0.05) and 2003 (P < 0.001), as well as in 2004 for conventional till (P <
0.001). Quadratic N responses for the V-blade application method occurred
at Lahoma for no-till in 2001 (P < 0.01), 2002 (P < 0.05), and 2004 (P <
0.05), but not for conventional till except in 2003 (P < 0.05). Although
not specifically measured, increased N immobilization was likely present in
no-till plots as in previous research (Smith and Sharpley, 1990; Knowles et
al., 1993). Highly significant interactions were found between tillage and
N rate in 2001 (P < 0.001), 2002 (P < 0.01), and 2004 (P < 0.05) at Lahoma.
The lack of an interaction at the Efaw site in all four years would imply
that immobilization did not greatly affect N availability for wheat
production, but wheat response to N was high in 2003 and 2004 as were grain
yields. This indicates that N availability was only limited at Efaw during
high yielding years possibly due to limited sinks of plant available N in
the soil.
Nitrogen Use Efficiency
Nitrogen
use efficiency was generally low for the first three years at Efaw and the
first two years at Lahoma. At Efaw, NUE was significantly higher for
no-till in 2003 when compared to conventional till (Table 5). Nitrogen use
efficiency was significantly higher at Lahoma for the no-till in 2001, but
in 2003 the conventional till was higher (Table 6). Although at Lahoma NUE
response to conventional tillage was high for both application methods in
2003 and for the knife application method in 2004, no NUE response to N was
observed for the V-blade application method in 2004. This indicates that
experimental error effected NUE response to N for the V-blade application
method and created the false-positive result of no-till significantly
improving NUE in 2004. At Efaw, the knife application method improved NUE
over the V-blade in no-till the initial year (P = 0.002), but there were no
significant differences in NUE between the AA application methods in either
tillage practice the following years. The V-blade applicator increased NUE
over knife applied AA at Lahoma in the no-till during the 2001 (P = 0.011),
2002 (P < 0.001), and 2004 (P < 0.1) crop years.
A NUE quadratic
response to N with the knife applicator was observed in the no-till during
the 2003 crop year at Efaw (P < 0.02). At Lahoma, negative linear N
responses to NUE occurred with the knife applicator in conventional till
during the 2002 (P < 0.05), 2003 (P < 0.1), and 2004 (P < 0.01) crop years.
Negative linear NUE responses to N were observed with the V-blade applicator
at Lahoma in conventional till the first three years (P < 0.05) and in
no-till during the 2001 (P < 0.01), 2002 (P < 0.05), and 2004 (P < 0.05)
crop years. Significant interaction between tillage and N method was
detected the initial year at Efaw (P < 0.001) as well as in 2002 (P < 0.001)
and 2004 (P < 0.1) at Lahoma, further revealing that tillage practices
affected applicator efficiency.
Additional Parameters
In light of
conflicting results being evaluated for the first two years of data
collection, additional parameters were measured starting in 2003. Of the
four site years of residue coverage data, a significant difference was only
found between the AA applicators at Efaw in 2003 where the knife applicator
caused less disturbance than the V-blade (data not shown). Furthermore,
residue disturbance averaged less than fifteen percent each year. However,
while the modified, line-intersect method prevented potential bias and
inaccuracy from using a visual estimate of residue coverage and disturbance,
the modified method limited accuracy to plus or minus five percent. As a
result, small differences in residue disturbance that occurred between the
AA applicators may have been overlooked.
Significant
differences in plant population were noted for tillage and N method at Efaw
in 2003 with the no-till treatments having higher plant populations than the
conventional till and V-blade application method having higher plant
populations in both tillage practices. However, a linear relationship
failed to exist between plant population and residue coverage at Efaw in
2003 and no significant differences were found at Efaw in 2004 or either
year (2003-2004) at Lahoma (data not shown). This data indicates that while
plant population was influenced by AA application method at Efaw in 2003, an
additional soil characteristic other than residue coverage was improved by
the V-blade applicator over the knife. Furthermore, no significant
differences were found between the AA applicators for grain yield or NUE at
Efaw in 2003, signifying that some other effect has caused the V-blade
advantage since plant population did not influence either grain yield or
NUE.
Bulk
density was considerably lower at the Lahoma site compared to Efaw, but no
significant differences in bulk density were found in the treatments at
either location (data not shown). At Lahoma, the knife applicator had
greater soil compaction between the 0-15 and 25-30 cm depths in the no-till
and at the 7.5 and 22.5-25 cm depths in the conventional till compared to
the V-blade (Figure 1). No significant differences resulted between the
tillage systems at Lahoma. However, at Efaw no-till significantly reduced
soil compaction at the 0-5 and 15-20 cm depths (Figure 2). Furthermore, the
knife applicator had greater soil compaction between the 2.5-12.5 and
27.5-30 cm depths in no-till at Efaw compared to the V-blade, but no
differences were found between the AA applicators in conventional till.
No-till crop production reduced soil compaction at Efaw and the V-blade
applicator reduced soil compaction within the no-till at both locations, but
NUE was only improved with the V-blade in no-till at Lahoma and no-till only
improved NUE at Efaw one of four years. However, across both tillage
systems, the Efaw soil penetrometer data confirms the presence of a plow-pan
or root-limiting layer between 1the 2.5 and 17.5 cm depths, characteristic
of intensively tilled soil (Figure 2). Potentially this explains the
limited effectiveness of the V-blade at Efaw and a possible component of the
generally lower grain yields observed at Efaw compared to Lahoma. Soil
compaction was measured by soil resistance in this study, which, as reported
by Vaz et al. (2001), does not always relate well to bulk density due to
multiple variables that influence soil resistance that does not necessarily
affect bulk density.
CONCLUSIONS
Although the no-till
system showed comparable grain yield at two site years and significantly
higher in a third compared to conventional tillage, the latter had a
distinct advantage in grain yield over the four-year duration of this
study. Over time N response in conventional till increased tremendously
along with grain yield and eventually exceeded no-till. Furthermore, the 61
kg ha-1 N rate appeared optimum in conventional till while the
123 kg ha-1 N rate appeared optimum in no-till, suggesting that
no-till required higher additional N to maximize grain yield. The V-blade
application method improved grain yield and NUE in no-till at one site,
potentially due to reduced soil compaction, but neither AA applicator showed
an advantage in conventional tillage. Previous crop residue disturbance
averaged less than 15% for both AA applicators all four site years and
residue disturbance did not influence the AA application effects.
Mid-season plant populations taken during the 2003 and 2004 crop years were
insignificant in three of the four site years and plant population did not
influence grain yield and NUE. Although four years of data were collected
in this study, additional data may be needed to properly evaluate no-till
cropping systems. Over the four years of this study no-till reduced soil
compaction and conventional tillage produced higher grain yields, but no
conclusive advantages were found in NUE for either tillage practice or AA
application method.
REFERENCES
Aase, J.K.
and J.L. Pikul. 1995. Crop and soil response to long-term tillage
practices in
the northern Great Plains. Agron. J. 87:652-656.
Bauer, A.
and A.L. Black. 1994. Quantification of the effect of soil organic matter
content on
soil productivity. Soil Sci. Soc. Am. J. 58:185-193.
Bonfil, D.J.,
I. Mufradi, S. Klitman, and S. Asido. 1999. Wheat grain yield and soil
profile
water distribution in a no-till arid environment. Agron. J.
91:368-373.
Bouman, O.T.,
D. Curtin, C.A. Campbell, V.O. Biederbeck, and H. Ukrainetz.
1995. Soil
acidification from long-term use of anhydrous ammonia and urea. Soil Sci.
Soc. Am. J. 59:1488-1494.
Cantero-Martinez, C., G.J. O’Leary, and D.J. Connor. 1999. Soil water and
nitrogen
interaction in wheat in a dry season under a fallow-wheat cropping system.
Australian Journal of Experimental Agriculture 39:29-37.
Christensen,
N.B., W.C. Lindemann, E. Salazar-Sosa, and L.R. Gill. 1994.
Nitrogen and
carbon dynamics in no-till and stubble mulch tillage systems. Agron. J.
86:298-303.
Dao, T.H.
1993. Tillage and winter wheat residue management effects on water
infiltration
and storage. Soil Sci. Am. J. 57:1586-1595.
Dick, W.A.
1983. Organic carbon, nitrogen, and phosphorus concentrations and
pH in soil
profiles as affected by tillage intensity. Soil Sci. Soc. Am. J.
47:102-107.
Doran, J.W.
1980. Soil microbial and biochemical changes associated with
reduced
tillage. Soil Sci. Soc. Am. J. 44:765-771.
Eck, H. V.
and O.R. Jones. 1992. Soil nitrogen status as affected by tillage,
crops, and
crop sequences. Agron. J. 84:660-668.
Edwards,
W.M., M.J. Shipitalo, L.B. Owens, and L.D. Norton. 1990. Effect of
Lumbricus
terrestris L. burrows on hydrology of continuous no-till corn
fields. Geoderma 46:73-84.
Eghball, B.,
L.N. Mielke, D.L. McCallister, and J.W. Doran. 1994. Distribution of
organic
carbon and inorganic nitrogen in soil under various tillage and crop
sequences. J. Soil Water ConServ. 49:201-205.
Fox, R.H.
and V.A. Bandel. 1986. Nitrogen utilization with no-tillage. Pp. 117-148.
In
M.A. Sprague
and Triplett (eds.) No-tillage and surface tillage agriculture, the
tillage revolution.
John Wiley and Sons, New York.
Halvorson,
A.D., A. L. Black, J.M. Krupinsky, and S. D. Merrill. 1999.
Dryland
winter wheat response to tillage and nitrogen within an annual cropping
system. Agron. J. 91:702-707.
Hamid, A.
and R.L. Mahler. 1994. The potential for volatilization losses of
applied
nitrogen fertilizers from northern Idaho soils. Commun. Soil. Sci. Plant
Anal. 25(3&4), 361-373.
Ismail, I.,
R.L. Blevins, and W.W. Frye. 1994. Long-term no-tillage effects on soil
properties
and continuous corn yields. Soil Sci. Soc. Am. J. 58:193-198.
King, K.W.,
D.C. Flanagan, L.D. Norton, and J.M. Laflen. 1995. Rill erodibility
parameters
influenced by long-term management practices. Trans. ASAE
38:159-164.
Knowles,
T.C., B.W. Hipp, P.S. Graff, and D.S. Marshall. 1993. Nitrogen nutrition
of rainfed
winter wheat in tilled and no-till sorghum and wheat residues. Agron. J.
85:886-893.
Lal, R.,
A.A. Mahboubi, and N.R. Fausey. 1994. Long-term tillage effect and
rotation
effects on properties of a central Ohio soil. Soil Sci. Soc. Am. J.
58:517-522.
Lamb, J.A.,
G.A. Peterson, and C.R. Fenster. 1985. Wheat-fallow tillage systems
‘effect on
newly cultivated grassland soils’ nitrogen budget. Soil Sci. Soc. Am. J.
49:352-356.
McGregor,
K.C., C.K. Mutchler, and R.K. Cullum.1992. Soil erosion effects on
soybean
yields. Trans. ASAE 35:1521-1525.
McGregor,
K.C., R.F. Cullum, and C.K. Mutchler. 1999. Long-term management
effects on
runoff, erosion, and crop production. Trans. ASAE 42:99-105.
Merrill,
S.D., A.L. Black, and A. Bauer. 1996. Conservation tillage affects root
growth of
dryland spring wheat under drought. Soil Sci. Am. J. 60:575-583.
Morrison,
Jr., J.E., R.W. Rickman, D.K. McCool, and K.L. Pfeiffer. 1997. Measurement
of wheat
residue cover in the Great Plains and Pacific Northwest. J. Soil and
Water Cons. 52(1):59-65.
Norwood, C.
1994. Profile water distribution and grain yield as affected by
cropping
system and tillage. Agron. J. 86:558-563.
Oklahoma
Department of Agriculture. 2005. Personal communication. Oklahoma
Department of Agriculture, Oklahoma City, OK.
Peterson,
G.A., A.J. Schlegel, D.L. Tanaka, and O.R. Jones. 1996. Precipitation
use
efficiency as affected by cropping and tillage systems. J. Prod. Agric.
9:180-186.
Rao, S.C.
and T. H. Dao. 1996. Nitrogen placement and tillage effects on dry
matter and
nitrogen accumulation and redistribution in winter wheat. Agron. J.
88:365-371.
Rodriguez,
M.B. and N. Giambiagi. 1995. Denitrification in tillage and no-tillage
Pampean
soils: relationships among soil water, available carbon, and nitrate and
nitrous oxide production. Commun. Soil. Sci. Plant Anal.
26:3205-3220.
SAS
Institute. 1990. SAS/STAT user’s guide. Release 6.03 ed. SAS Inst.,
Cary,
NC.
Schepers,
J.S., D.D. Francis, and M.T. Thompson. 1989. Simultaneous determination
of total C,
total N, and 15N on soil and material. Commun. Soil Sci.
Plant Anal. 20(9&10):949-959.
Smith, S.J.
and A.N. Sharpley. 1990. Soil nitrogen mineralization in the
presence of
surface and incorporated crop residues. Agron. J. 82:112-116.
Smith, S.J.,
A.N. Sharpley, J.W. Naney, W.A. Berg, and O.R. Jones. 1991. Water
Quality
impacts associated with wheat culture in the southern Plains. J.
Environ. Qual. 20:244-249.
Swart, C.L.,
L.S. Murphy, and C.W. Swallow. 1971. Retention patterns and
effectiveness of anhydrous ammonia applied with an undercutting blade.
Agron. J. 63:881-884.
Tomar, J.S.
and R.J. Soper. 1981. Fate of tagged urea N in field with different
methods of N
and organic matter placement. Agron. J. 73:991-995.
Vaz, C.M.P.,
L.H. Bassoi, and J.W. Hoppans. 2001. Contribution of water content and
bulk density
to field soil penetration resistance as measured by a combined cone
penetrometer-TDR probe. Soil Till. Res. 60:35-42.
Vyn, T.J.
and B.A. Raimbault. 1993. Long-term effect of five tillage systems on
corn
response and soil structure. Agron. J. 85:1074-1079.
Weersink,
A., M. Walker, C. Swanton, and J.E. Shaw. 1992. Costs of
conventional
and conservation tillage systems. J. Soil and Water Cons.
47:328-334.
Wienhold,
B.J. and A.D. Halvorson. 1999. Nitrogen mineralization responses to
cropping,
tillage, and nitrogen rate in the northern great plains. Soil Sci. Soc.
Am. J. 63:192-196.
Received:
Revised:
Accepted :
Table 1. Initial surface
(0-15 cm) and sub-soil (15-30 cm) test results prior to experiment initiation at
Efaw and Lahoma OK.
Sample |
NH4-N |
NO3-N |
P |
K |
pH |
-------------------------- mg kg-1 ---------------------- |
Lahoma (0-15 cm) |
14 |
9 |
9 |
282 |
5.7 |
Lahoma (15-30 cm) |
16 |
4 |
6 |
222 |
6.2 |
Efaw (0-15 cm) |
16 |
11 |
28 |
225 |
5.7 |
Efaw (15-30 cm) |
14 |
7 |
7 |
190 |
6.4 |
NH4-N and NO3-N
– 2 M KCL extract; P and K – Mehlich-3 extraction; pH – 1:1 soil:deionized
water
Table 2. Planting,
fertilizer, and harvest dates at Efaw and Lahoma, OK, 2000-04.
Location |
Crop Year |
Planting |
Fertilizer
Application |
Grain Harvest |
Efaw |
2000-2001 |
11-30-00† |
11-22-00 |
6-11-01 |
2001-2002 |
10-01-01 |
9-11-01 |
6-21-02 |
2002-2003 |
10-17-02 |
9-03-02 |
6-23-03 |
2003-2004 |
9-27-03 |
9-18-03 |
6-15-04 |
Lahoma |
2000-2001 |
11-27-00 |
11-27-00 |
6-14-01 |
2001-2002 |
10-03-01 |
9-04-01 |
6-25-02 |
2002-2003 |
10-08-02 |
9-06-02 |
6-17-03 |
2003-2004 |
10-16-03 |
9-19-03 |
6-12-04 |
†
month-day-year format
Table 3.
Grain yield treatment means at Efaw, OK, 2001-2004.
Treatment |
2001 |
2002 |
2003 |
2004 |
Avg. |
Tillage |
App/
Source |
N rate
kg N ha-1 |
Grain yield
------------------------
(Mg ha-1) ---------------------------- |
CT |
Knife |
0 |
2.26 |
3.57 |
3.40 |
3.03 |
3.12 |
61 |
2.27 |
3.59 |
3.82 |
4.18 |
3.46 |
123 |
2.50 |
3.80 |
3.78 |
4.48 |
3.64 |
185 |
2.53 |
3.64 |
3.68 |
4.98 |
3.71 |
Avg. |
|
2.39 |
3.65 |
3.67 |
4.17 |
3.49 |
V-blade |
0 |
2.13 |
3.83 |
2.67 |
2.34 |
2.58 |
61 |
2.48 |
3.73 |
3.89 |
4.29 |
3.60 |
123 |
2.63 |
3.99 |
3.84 |
4.96 |
3.85 |
185 |
2.61 |
3.76 |
3.87 |
4.91 |
3.79 |
Avg. |
|
2.51 |
3.85 |
3.57 |
4.13 |
3.51 |
NT |
Knife |
0 |
1.90 |
2.98 |
2.67 |
2.48 |
2.57 |
61 |
2.29 |
3.42 |
3.50 |
3.56 |
3.19 |
123 |
2.55 |
3.66 |
3.04 |
4.54 |
3.50 |
185 |
2.66 |
3.81 |
3.50 |
4.81 |
3.67 |
Avg. |
|
2.35 |
3.43 |
3.18 |
3.85 |
3.23 |
V-blade |
0 |
2.10 |
2.94 |
2.70 |
2.49 |
2.53 |
61 |
2.11 |
3.11 |
3.23 |
3.13 |
2.87 |
123 |
2.48 |
3.25 |
3.40 |
4.40 |
3.38 |
185 |
2.44 |
3.67 |
3.56 |
4.78 |
3.61 |
Avg. |
|
2.28 |
3.24 |
3.22 |
3.70 |
3.12 |
SED |
0.13 |
0.27 |
0.12 |
0.36 |
--- |
|
|
|
|
|
|
|
|
|
SED is the standard error
of the difference between two equally replicated means.
CT= conventional tillage; NT=
no-till
Table 4.
Grain yield treatment means at Lahoma, OK, 2001-2004.
Treatment |
2001 |
2002 |
2003 |
2004 |
Avg. |
Tillage |
App/ Source |
N rate
kg N ha-1 |
Grain Yield
----------------------- (Mg ha-1)
----------------------------- |
CT |
Knife |
0 |
1.77 |
3.98 |
3.63 |
2.25 |
2.97 |
61 |
2.13 |
4.29 |
4.07 |
4.51 |
3.75 |
123 |
1.29 |
3.92 |
4.71 |
4.31 |
3.56 |
185 |
2.07 |
3.86 |
4.48 |
3.98 |
3.60 |
Avg. |
|
1.82 |
4.01 |
4.22 |
3.66 |
3.48 |
V-blade |
0 |
1.90 |
3.74 |
2.28 |
4.24 |
3.15 |
61 |
2.45 |
4.07 |
4.31 |
4.27 |
3.73 |
123 |
1.70 |
3.80 |
4.50 |
4.04 |
3.51 |
185 |
1.97 |
3.94 |
4.65 |
4.15 |
3.68 |
Avg. |
|
2.01 |
3.91 |
3.93 |
4.18 |
3.53 |
NT |
Knife |
0 |
1.12 |
2.50 |
3.87 |
2.46 |
2.56 |
61 |
1.43 |
2.92 |
5.03 |
3.48 |
3.16 |
123 |
1.54 |
2.97 |
5.09 |
3.71 |
3.33 |
185 |
1.87 |
2.60 |
4.53 |
3.94 |
3.24 |
Avg. |
|
1.49 |
2.79 |
4.63 |
2.86 |
3.10 |
V-blade |
0 |
0.78 |
2.62 |
4.64 |
1.39 |
1.85 |
61 |
1.68 |
3.33 |
4.80 |
3.10 |
3.10 |
123 |
1.89 |
3.62 |
4.96 |
3.78 |
3.56 |
185 |
1.66 |
3.59 |
5.13 |
3.63 |
3.50 |
Avg. |
|
1.50 |
3.32 |
4.97 |
2.89 |
3.11 |
SED |
0.31 |
0.20 |
0.42 |
0.46 |
--- |
|
|
|
|
|
|
|
|
|
SED is the standard error
of the difference between two equally replicated means.
CT= conventional tillage; NT=
no-till
Table 5.
Nitrogen Use Efficiency treatment means at Efaw, OK, 2001-2004.
Treatment |
2001 |
2002 |
2003 |
2004 |
Avg. |
Tillage |
App/ Source |
N rate
kg N ha-1 |
------------------------- NUE
(%) ----------------------- |
CT |
Knife |
61 |
5 |
4 |
15 |
68 |
23 |
123 |
10 |
8 |
13 |
30 |
15 |
185 |
6 |
6 |
4 |
41 |
14 |
Avg. |
|
7 |
6 |
10 |
46 |
17 |
V-blade |
61 |
22 |
16 |
15 |
74 |
31 |
123 |
15 |
13 |
11 |
61 |
25 |
185 |
11 |
6 |
10 |
45 |
18 |
Avg. |
|
16 |
12 |
12 |
60 |
25 |
NT |
Knife |
61 |
21 |
21 |
39 |
47 |
32 |
123 |
23 |
18 |
10 |
39 |
24 |
185 |
16 |
12 |
17 |
37 |
21 |
Avg. |
|
20 |
17 |
23 |
41 |
26 |
V-blade |
61 |
4 |
5 |
32 |
36 |
18 |
123 |
10 |
13 |
25 |
38 |
21 |
185 |
7 |
20 |
20 |
43 |
23 |
Avg. |
|
7 |
13 |
26 |
39 |
21 |
SED |
5 |
11 |
12 |
17 |
--- |
NUE= (Grain N uptake of N
treatment – Grain N uptake of check) / N rate
SED is the standard error of
the difference between two equally replicated means.
CT= conventional tillage; NT=
no-till
Table 6. Nitrogen Use
Efficiency treatment means at Lahoma, OK, 2001-2004.
Treatment |
2001 |
2002 |
2003 |
2004 |
Avg. |
Tillage |
App/ Source |
N rate
kg N ha-1 |
------------------------- NUE
(%) ----------------------- |
CT |
Knife |
61 |
14 |
23 |
46 |
90 |
43 |
123 |
0 |
13 |
40 |
50 |
24 |
185 |
6 |
6 |
28 |
36 |
19 |
Avg. |
|
5 |
14 |
38 |
59 |
29 |
V-blade |
61 |
29 |
23 |
64 |
20 |
36 |
123 |
3 |
1 |
32 |
0 |
8 |
185 |
5 |
4 |
25 |
3 |
9 |
Avg. |
|
12 |
11 |
40 |
6 |
17 |
NT |
Knife |
61 |
19 |
14 |
36 |
58 |
27 |
123 |
13 |
13 |
39 |
45 |
27 |
185 |
14 |
4 |
22 |
37 |
19 |
Avg. |
|
15 |
10 |
32 |
43 |
24 |
V-blade |
61 |
44 |
48 |
39 |
78 |
53 |
123 |
24 |
35 |
27 |
72 |
39 |
185 |
16 |
28 |
24 |
45 |
27 |
Avg. |
|
28 |
37 |
29 |
65 |
40 |
SED |
7 |
9 |
10 |
14 |
--- |
NUE= (Grain N uptake of N
treatment – Grain N uptake of check) / N rate
SED is the standard error of
the difference between two equally replicated means.
CT= conventional tillage; NT=
no-till
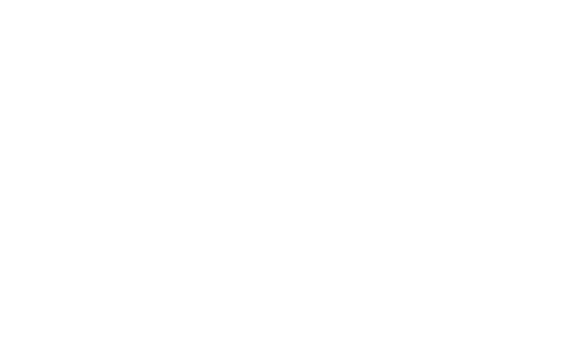
Figure 1. Effect of tillage
and N method on soil compaction over four years for depths 0-30 cm at Lahoma,
OK. SED is the standard error of the difference between two equally replicated
means. SED by depth from 0 to 30 cm with an interval of 2.5 cm is 156, 200, 241,
262, 292, 307, 278, 297, 327, 331, 332, 341, and 348.
CT= conventional tillage; NT=
no-till
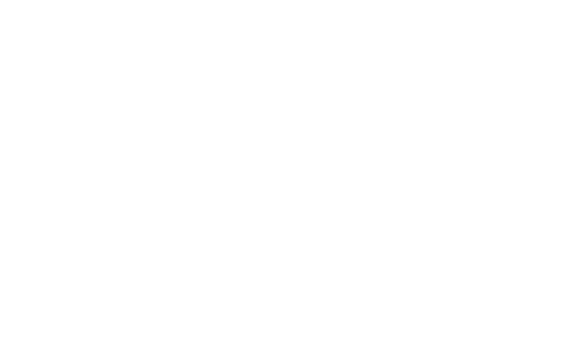
Figure 2. Effect of tillage
and N method on soil compaction over four years for depths 0-30 cm at Efaw, OK.
SED is the standard error of the difference between two equally replicated means
SED by depth from 0 to 30 cm with an interval of 2.5 cm is 165, 200, 212, 222,
280, 283, 291, 241, 190, 190, 205, 207, and 199.
CT= conventional tillage; NT=
no-till |